How to Win the War Against Infection, Inflammation, Disease and Immunity
New Jersey Medical School researchers describe the rapid battlefield tests taking shape at the Institute for Infectious and Inflammatory Disease

It’s a scary struggle. Forget guns, bullets, bombs and drones. Humans are entangled in a far more lethal world war than the conflicts often described in the news. The numbers and potential kill factors are frightening. Ebola is just one recent enemy amidst many. Every year, two million people develop hospital-acquired infections, for example, and existing antibiotics are often useless.
The good news: When it comes to fighting the battle against infection, inflammation, disease and immunity gone haywire, there are new weapons in the research labs. Let’s talk about helminth worms, for instance, or enzymes with crab claws to unhinge bacteria, and secrets in dirt. Let’s also applaud the rapid battlefield tests being designed by New Jersey Medical School researchers. There is an overall plan taking shape with strategic headquarters at the Institute for Infectious and Inflammatory Disease (I3D) on the NJMS campus in Newark.
“It’s time to join forces to tackle disease at the intersection of inflammation and infection,” says one of the directors of I3D, William C. Gause, senior associate dean of research. Born two years ago, the Institute has brought world-class scientists together to build upon the expertise at cutting-edge facilities like the Public Health Research Institute (PHRI), the Center for Immunity and Inflammation (CII) and the Center for Emerging and Re-Emerging Pathogens. “We are at the crossroads of discovery with unprecedented opportunity to develop new treatments for a wide range of deadly diseases,” according to Gause. “What if a simple diagnostic test could rapidly detect the world’s most contagious diseases? What if the body’s own immunological defenses could be harnessed to combat inflammation without compromising our resistance to disease?”
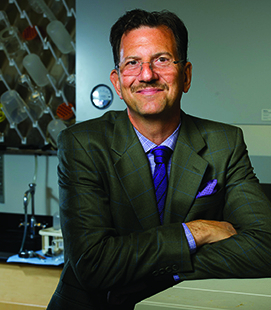
Inside the Antibiotic Labyrinth
Perhaps no one has seen more of this war upfront than David Perlin, executive director of PHRI. Last year, the National Institute of Allergy and Infectious Diseases (NIAID) and its Centers of Excellence for Translational Research (CETR) chose Perlin as the principal investigator and awarded him $26 million to develop new antibiotics. Always straightforward, he says, “We have a worldwide crisis with major unmet needs. There aren’t sufficient drugs and too many are in the same chemical classes that make them resistant to just about everything. The pipeline for new antibiotics is running at a trickle. In fact, the medicine cabinet is empty in many cases.”
A combination of factors led the world into this war-with-no-weapons bind. Pharmaceutical companies once built their businesses on medications for acute conditions and have not made new antibiotic development a priority for decades because it’s not profitable enough. “When you get sick, you take an antibiotic for 10 to 12 days at most. That’s it,” Perlin explains. “Chronic conditions like high blood pressure or cardiac disease require drugs long-term, where the real money is.” Meanwhile, bacteria continue to develop resistance so the need for new antibiotics is ongoing. The Food and Drug Administration (FDA) also set the bar for drug approval too high requiring years of clinical trials not for safety but for efficacy, a rule that has finally been changed for antibiotic-resistant conditions. “Why would a company spend a billion dollars and 10 to 15 years on a drug that only affords them three to four years of U.S. patent protection?” Perlin asks.
“To jumpstart the drug discovery process,” Perlin has five projects with six core areas of expertise, in a new “Center to Develop Therapeutic Countermeasures to High-threat Bacterial Agents.” War-like words like “countermeasures” and “high-threat” are used on purpose, he explains, because “we want drugs that are novel and could potentially be used for strategic national purposes. It’s a battle against these pathogens.”
By choosing key players with deep experience at each point in the drug “discovery to market” process, including Cubist, an antibiotic pharmaceutical company, he has put together a “group much like an academic based pharma company. This could become the paradigm for how to do early phase drug discovery especially in areas of unmet needs.”
A significant component of this grant involves researchers from the Center for Emerging and Re-Emerging Pathogens run by David Alland. Alland focuses on different aspects of Mycobacterium tuberculosis biology, epidemiology, and rapid diagnostics, and his group is looking at novel targets to develop new anti-TB drugs. He designed the new test for TB endorsed by the World Health Organization in 2010 and is now working on one for Ebola after receiving $640,000 from the NIH.

Other NJMS researchers have a variety of backgrounds. From medicinal chemistry (Joel Freundlich), pharmacokinetics (Veronique Dartois), structural refinement (Min Lu), in vitro screening (Nancy Connell), to the sophisticated animal infection modeling in Perlin’s own domain, “we can move compounds through rapidly,” Perlin explains. A separate board includes prominent pharmaceutical executives “to help us prioritize. Imagine if you are generating thousands of compounds. You need advice picking the ones the pharmaceutical world would deem appropriate to develop.” The aim is to license these discoveries out to companies. “Academic scientists are good at discovering small molecules but they don’t have the resources to do the compound refinement” or the ability to carry out small and large animal studies, as a prelude to human clinical trials.
“Dirty” Secrets and Crab Claw Enzymes
At the early end of discovery is Sean Brady from Rockefeller University. “I’ve known Sean for years and he has really cool, innovative research,” Perlin explains. “He’s utilizing nature, bumping it up to go faster.” Actually, Brady is looking for drugs in dirt, sifting soil samples for uncultured, novel bacteria that could lead to antibiotics. “Genetic evidence hints there are many more bacteria out there,” Brady believes, with “vast chemical and biosynthetic potential.” His Laboratory of Genetically Encoded Small Molecules uses gene sequencing to synthesize “a rich, undiscovered source of useful natural products.” Historically speaking, he’s on the right track. Many current antibiotics, including tetracycline and vancomycin as well as cancer drugs and immune therapeutics, have roots right in nature.
What if the answer to the antibiotic crisis was hidden in plain sight? Besides leading the medicinal chemistry area, Freundlich is focused on learning from hundreds of thousands of molecules that have already been tested for antibacterial activity. The data resides in publically accessible electronic archives, dormant for years until he and longtime collaborator, Sean Ekins demonstrated how computational models can leverage this information to predict new antibacterial agents.
Then there is Richard Ebright, from Rutger’s Waksman Institute of Microbiology, where the groundbreaking antibiotic streptomycin was developed in 1943. He “is looking at a promising molecular target that can overcome existing antibiotic resistance,” Perlin explains. “He has the right kinds of molecules to jump the divide from preclinical to clinical.” Three compounds interfere with the action of bacterial RNA polymerase, an enzyme with a “shape reminiscent of a crab claw,” Ebright says. It uses a “pincer hinge” to stop bacteria from obtaining DNA genetic information.
Worms and Immunity
Asking big questions and digging deep for answers to immunity and inflammation come naturally for Gause, an intense, no-nonsense researcher whose lab interests have focused on how worms, helminths, trigger immune switches.
“Worms?”
Yes, this is metazoan parasite theory. “This research could be important in the development of vaccines against a variety of pathogens,” he explains. Helminths have coevolved with vertebrates for millions of years, shaping the activation and function of immune responses. To understand them in the lab is key to finding answers to many mysteries of immunity. When helminths and other metazoan parasites enter, exit, or migrate through their host, macrophages (disease-fighting, white blood cells) are activated in defense. Then, in a complex, type 2, trained immune response, the second time the host is exposed to the parasite—in the same way a vaccine works—the macrophage remembers just what to do. Lesson learned, the host survives, stronger than before, immunological memory bumped up. “If our own body’s macrophages can be effectively trained to fight invaders for which traditional vaccines are ineffective, they could destroy viral invaders like HIV or Ebola,” he explains. This differs from type 1, innate immunity where the body launches a vast array of defenses against infectious agents or invading pathogens, sending phagocytic cells like macrophages and neutrophils into battle, sometimes with unwanted side effects from toxic antimicrobial products.
Gause has found another piece to this immune puzzle through his worm work. The type 2 immune response triggered by helminths produces cytokines and factors that are different from the harmful inflammation of innate immunity that can lead to a variety of diseases and conditions including: cardiovascular disease, diabetes, implant failure, chronic wounds, inflammatory disease, and even obesity. In fact, this type 2 immunity has regulatory cells that block bad inflammation associated with type 1 immune response. It also can directly enhance wound healing and tissue repair. Harnessing components of the type 2 immune response to control harmful inflammation and enhance wound healing is an active area of study in the Gause laboratory.
Breakthrough Tests
In a perfect example of the kind of collaboration needed to win any war against pathogens, two researchers have been reaching across laboratory lines for a breakthrough test for Lyme disease. Victims are already applauding because up until now, the science helping them has been inadequate. The ticks and bacteria have been winning.

At the conference table on the third floor of PHRI, the air of excitement is palpable. Nikhat Parveen is an associate professor in the Department of Microbiology, Biochemistry and Molecular Genetics who studies tick-borne pathogens, syphilis and multi-drug resistant bacteria like Pseudomonas aeruginosa. Salvatore A. E. Marras, an assistant professor in the same department, shares a PHRI lab on the other side of the building with Fred Kramer and Sanjay Tyagi, the team that invented molecular beacons 20 years ago. Together, Parveen and Marras have designed a sensitive, multiplex test not only for Borrelia burgdorferi, the spiral-shaped bacteria transmitted by ticks that cause Lyme disease, but also for two other tick-transmitted pathogens that coinfect the critters and wreak havoc in humans: Anaplasma phagocytophilum, an intracellular bacterium, and Babesia microti, a parasite similar to what causes malaria. Both are dangerous and often undetected.
“All three pathogens use white-footed mice as a reservoir host and white-tailed deer populations to spread long distances. Babesia grows in red blood cells and the infection may remain asymptomatic. Anaplasma thrives in white blood cells. Both can lead to severe, fatal disease,” Parveen explains. “These two pathogens can also be transmitted by blood transfusions but it is difficult to check for them.” And, because infected patients don’t always feel sick in early stages, they have been allowed to donate blood, endangering the supply. Blood banks aren’t testing for Babesia and Anaplasma because efficient, cost effective diagnostic assays haven’t been available.
Marras relishes teamwork. “This is so mutually beneficial. The introduction of molecular beacons has made it possible to detect RNA and DNA molecules in living cells and in clinical samples to quantify and detect multiple pathogens simultaneously.” Kramer and Tyagi describe the probes like this: “Imagine a magic reagent to which is added a droplet of body fluid from a patient. A glow appears in the tube and the color of the glow identifies the pathogen responsible for the patient’s illness.”
News of this test has already created excitement among patients. “You would not believe the overwhelming response to our first paper in 2009. We were still editing, but because the manuscript was online,” Parveen recalls, “I was getting calls from everywhere. These people are suffering and want answers so our assay is very timely.”
The Centers for Disease Control and Prevention (CDC) estimates that only one-tenth of Lyme disease cases are accurately diagnosed. Clinical manifestations are tricky and testing hasn’t been effective, unable to distinguish between active and underlying disease. And since antibodies against the pathogens persist in blood for a long time, it has even been difficult to confirm a cure. Patients are also susceptible to secondary infections that might explain some of the random symptoms reported in Lyme disease.
What’s next? They have applied for a patent and are speaking with a Massachusetts company who is ready to start developing the assay for field application, particularly for the blood bank samples. Parveen plans more human blood testing and is working with physicians like Kristine Gedroic, a Harvard-trained specialist who has Lyme disease patients in her integrative medical practice in Morristown; Kathleen Casey of Jersey Shore University Medical Center; Melvin Weinstein, Chief of Infectious Diseases, Allergy and Immunology, Robert Wood Johnson Medical School (RWJMS); and Tanaya Bhowmick, RWJMS. Finding more patient samples from the Northeastern U.S. and Europe where tick-borne diseases are prevalent is on Parveen’s to-do list. She also wants to expand her technical expertise to develop assays for early detection of emerging multi-drug resistant Gram-negative bacteria in hospitalized patients and for efficient diagnosis of syphilis.
Parveen says, “Our test will be able to diagnose from early to chronic stages of Lyme as well as babesiosis and anaplasmosis in one assay. It is so sensitive and specific that any level of the pathogens present can be found.” Coinfections with these three pathogens have been increasing,” she adds. “There is a lot of tick-borne disease in Europe where Lyme originated hundreds of years ago though it was first diagnosed in the U.S. in Lyme, Connecticut. You see a lot of Lyme disease from Massachusetts to Maryland but not in Newark.”
What you do see in Newark, however, are world-class scientists advancing the war against infection, inflammation, and immune disorders.
This article is adapted from Pulse Magazine, New Jersey Medical School, Spring 2015.